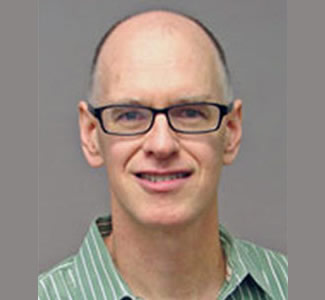
Donald Burke
Professor of Molecular Microbiology & Immunology
Biochemistry,Molecular Microbiology & Immunology,
burkedh@missouri.edu
(573) 884-1316
Fields of Interest
- Mechanism and evolution of catalysis by ribozymes.
Education
- Ph.D. 1992, University of California, Berkeley
Research Statement
Understand the mechanism and evolution of catalysis by ribozymes and the molecular basis of retroviral inhibition by RNA-based drugs.
Both aptamers and ribozymes are found in nature and can be isolated from combinatorial libraries containing ~1015 species. Ribozymes are RNA enzymes, while aptamers are RNAs selected to bind molecular partners. LIFE IS MOLECULAR RECOGNITION! By understanding, controlling, and manipulating the transient, non-covalent interactions that govern recognition, we can control the molecules of life. Our work is defining whether metabolic ribozymes can be engineered to replace protein enzymes inside cells, and whether aptamer inhibitors of HIV-1 replication can illuminate viral pathogenesis. We are also learning how the three-dimensional structure of a ribozyme directs catalysis and whether multi-clade targeting of aptamers prevents the evolution of drug resistant HIV.
Whether we are thinking about the Origin of Life, about the Molecular mechanisms of Viral Pathogenesis, or about RNA-based therapeutics, three kinds of questions underly our work:
- What can nucleic acids do?
- How do their sequences and structures relate to their ability to do it?
- Can we engineer new biologies by expressing artificial RNAs in cells?
Most of the RNA and ssDNA molecules we work with are isolated using the technology of in vitro directed molecular evolution (SELEX, Systematic Evolution of Ligands by EXponential enrichment). The SELEX technology recovers rare functional molecules – for almost any function – from exceedingly complex mixtures, allowing us to explore the chemical and biological limits of what RNA and DNA can do.
As new sequences become available, they will be posted to DARS, the Database of Aptamer and Ribozyme Sequences.
This site will soon be updated to reflect the lab’s current directions. In the meantime, here is what we have sent the last several years thinking about:
- Molecular recognition in RNA-protein and RNA-small molecule complexes, including development of nucleic acids as therapeutic agents;
- Catalytic RNA and ssDNA molecules;
- Using in vitro-selected RNAs to redirect cell metabolisms, with emphasis on metabolic engineering and modeling the origin and early evolution of Life.
RNA-protein and RNA-small Molecule Interactions
Life is molecular recognition! Macromolecules communicate with each other in part by forming specific, high-affinity complexes, as in mRNA localization, gene regulation, pre-mRNA splicing, and ribosome assembly. RNA-small molecule interactions are also important, as in antibiotic binding by ribosomal RNA and guanosine cofactor-binding by self-splicing RNAs. The central goal of this work is to understand the structural determinants of specificity in RNA-protein and RNA-small molecule interactions.
RNA-protein interactions and antiviral gene therapy agents. The Reverse Transcriptase (RT) protein of HIV is essential for viral replication. Anti-RT drugs such as AZT block viral replication, but their clinical effectiveness is limited by the rapid appearance of resistance mutations. Aptamers that bind HIV RT make numerous contacts with the protein, inhibit its activity, and offer an alternative to small-molecule drugs in the form of anti-viral gene therapy. Preliminary work along these lines in our collaborators’ labs shows great promise for this approach. Knowledge of RT’s ability to mutate into aptamer resistant forms is essential for designing proper candidates for clinical use, although the viability of such mutants and the frequency with which they might arise in a clinical setting are unknown. Therefore, we are using several aproaches to analyze the biochemical details of the RNA-protein interaction and to quantitate RT’s ability to mutate into resistant forms. The locations of aptamer-resistant mutations in the known crystal structure of the protein (454k jpeg image)will help in understanding the molecular basis for resistance.
What does an RNA binding pocket look like? RNA aptamers that bind small molecule targets are ideal for studies of RNA folding. We study the RNA sequence requirements and binding interfaces for several RNA-small molecule interactions, each of which has provided a wealth of information and surprises. For example, aptamers to S-adenosyl methionine (SAM) bind several other adenosine-containing cofactors, and they have beautiful structures. They have already been used in another lab to isolate self-kinasing RNA’s, and they may be broadly useful for isolating several classes of ribozymes. The sequence and specificity of the SAM-binding RNA motif are distinct from our aptamers to CoenzymeA, which present their own unusual structural features, even through the SAM- and CoA-binding RNAs both recognize the adenosine portion of their targets. Understanding the relationships among macromolecular sequence, st ructure, and function are central problems for structural biology and it is an underlying theme of our work.
RNA Catalysts
A fundamental quest of modern biochemistry is to understand how enzymes work and to embellish them with new properties. Recent work with enzymatically active nucleic acids shows that they have a catalytic potential rivaling that of proteins, but there is much that remains poorly understood. The state of the art in this field is to define the range of reactivities that can be expected from ribozymes, the mechanisms by which they function, and their capacity to participate in biology.
Expanding the scope of RNA catalysis. Ribozymes with novel properties can be identified in pools of random sequences using variations on the SELEX procedure. We are isolating ribozymes and deoxyribozymes for several classes of reactions. Of particular interest will be those that catalyze essential metabolic reactions normally carried out by protein enzymes. Additional projects are underway to examine the influence of temperature and metal ions on RNA function and on its in vitro evolution.
Evolution of catalysis through recombination and assembly. Accumulation of favorable point mutations during in vitro RNA evolution has been widely used to optimize existing ribozyme activities or to produce RNAs with altogether new activities. RNA should also be able to undergo evolution by recombining functional domains. To test this hypothesis, we have developed chimeric SELEX; which experimentally simulates random recombination among functional RNA domains. We are using it to build ribozymes from their component parts and to test the role of recombination in ribozyme evolution and engineering.
The Origin of Life & Metabolic Engineering
The RNA World Theory for the origin and early evolution of life postulates that ribozymes drove cell metabolisms before modern protein synthesis evolved. This theory was originally proposed to circumvent the “chicken-and-egg” paradox of how to initiate the flow of genetic information at life’s origin, since proteins encoded by DNA are required for the replication of DNA. The discovery of natural catalytic RNAs renewed vigorous speculation over the role of RNA in early evolution, most of it going well beyond the reach of supporting data. Through in vitro selection technologies, we can now test RNA World hypotheses through direct experimentation. We want to know whether ribozymes can catalyze a collection of reactions diverse enough to support life and how complex functions may have evolved (above), but we also want to know whether ribozyme catalysis can support life.
Is RNA-based life possible? If modern metabolism evolved from a ribozyme-driven metabolism, why did proteins ultimately come to dominate? To anwers these questions, we will replace essential cellular proteins with ribozymes, effectively creating model “ribo-organisms” that will help us better understand how such life forms may have functioned and evolved more than 3.8 billion years ago. What can we expect from an RNA-based metabolism, and what natural constraints might it experience? By replacing essential protein enzymes with ribozymes inside cells, we will in effect be reversing the course of evolution while defining the viability of RNA-based life forms.
Using aptamers and ribozymes to engineer new biologies. Recent years have seen a flood of reports showing how aptamers can be used to block the functions of extracellular, cytoplasmic, and nuclear proteins, with rationally predictable consequences for the cells in which they are expresesd. Nucleolytic ribozymes are also coming into maturity for cleaving target mRNA, and some of these ribozymes are entering clinical trials as anti-cancer agents. The potential for these applications balloons with the the number of new RNAs engineered in vitro. Being able to marry the technologies of in vitro selections and microbial genetics will lay the foundations for designing micro-organisms that can detoxify hazardous material, for gene therapy approaches to human metabolic diseases, and for a host of other applications.
No one can be truly successful in a vacuum, and we encourage collaborations with experts in areas other than our own. We have active collaborations with X-ray crystallographers to determine three-dimensional structure of some of these RNAs, with clinical physicians to develop aptamers with anti-inflammatory biomedical applications, with virologists to move the anti-HIV aptamers towards clinical application, with synthetic chemists to probe the effects of backbone modification on aptamer stability and nuclease resistance, and with inorganic spectroscopists to determine the role of metal ions in catalysis by catalytic RNA and DNA. This is an exciting time for nucleic acid research!
Publications
- HIV-related Publications, 2005-2012
- RNA Catalysis-related Publications, 2005-2012
- Other Recent Publications, 2002-2004
HIV-related Publications, 2005-2012
- Mark A. Ditzler, Margaret J. Lange, Chris Bottoms, Debojit Bose, Katherine Virkler, Andrew W. Sawyer, Angela S. Whatley, William Spollen, Scott Givan and Donald H. Burke. (2012) “New aptamers, neutral networks and next-generation sequencing: A fresh look at HIV reverse transcriptase aptamers. ” (in preparation).
- Margaret J. Lange, Tarun K. Sharma, Angela S. Whatley, Linda A. Landon, Michael A. Tempesta, Marc C. Johnson and Donald H. Burke. (2012) “Robust suppression of HIV replication by intracellularly expressed reverse transcriptase aptamers is independent of ribozyme processing.”
2012 Sep 4. doi: 10.1038/mt.2012.158. [Epub ahead of print]
- Donald H. Burke. (2012) “Cell-Penetrating RNAs: New Keys to the Castle” Invited Commentary for Mol. Ther. 2012 Feb; 20(2):251-3. doi:10.1038/mt.2011.306. The official link for this one is http://www.nature.com.proxy.mul.missouri.edu/mt/journal/v20/n2/full/mt2011306a.html, and the proofs for the paper are attached.
- Tanyaradzwa Ndongwe, Adeyemi Adedeji, Eleftherios Michailidis, Yee-Tsuei Ong, Atsuko Hachiya, Bruno Marchand, Emily Ryan, Devendra K. Rai, Karen A. Kirby, Shan-Lu Liu, Angela S. Whatley, Donald H. Burke, Marc Johnson, Ei-Ichi Kodama, Krista A. Delviks-Frankenberry, Vinay K. Pathak, Hiroaki Mitsuya, Michael A. Parniak, Kamal Singh & Stefan G. Sarafianos. (2012) “Biochemical, Inhibition, and Resistance Studies of Xenotropic Murine Leukemia Virus-Related Virus Reverse Transcriptase”#8221; Nucleic Acids Res. 40:345-359 (accepted August 2011). Supplemental.
- Mark A. Ditzler, Debojit Bose, Nikolozi Shkriabai, Bruno Marchand, Stefan G. Sarafianos, Mamuka Kvaratskhelia, and Donald H. Burke .(2011) “Broad-spectrum Aptamer Inhibitors of HIV Reverse Transcriptase Closely Mimic Natural Substrates” Nucleic Acids Research Oct., 39(18):83-237-47. Epub 2011 Jul 3. The official link for this one is http://nar.oxfordjournals.org/content/40/1/345.long, and the paper is attached. Supplemental.
- Mark A. Ditzler, Debojit Bose, Nikolozi Shkriabai, Bruno Marchand, Stefan G. Sarafianos, Mamuka Kvaratskhelia, and Donald H. Burke (2011) “Broad-spectrum Aptamer Inhibitors of HIV Reverse Transcriptase Closely Mimic Natural Substrates” Nucleic Acids Research Oct., 39(18):83=237-47. Epub 2011 Jul 3.
- Daniel Michalowski, Rebecca Chitima-Matsiga, Daniel M. Held and Donald H. Burke. (2008) “Novel bimodular DNA aptamers with guanosine quadruplexes inhibit hylogenetically diverse HIV-1 reverse transcriptases” Nucleic Acids Research 2008; doi:10.1093/nar/gkn891.
- Jay D. Kissel, Daniel M. Held & Donald H. Burke (2007) “Inhibition of reverse transcriptases by single-stranded DNA aptamers across HIV-1, SIVcpz and HIV-2 clades” AIDS Research & Human Retrovirology. 23:699-708.
- Daniel M. Held, Sarah Thacker, Daniel Michalowski, Jianfei Ji, Jay D. Kissel, John R. Rossi, Richard W. Hardy & Donald H. Burke (2007) “Differential inhibition of recombinant, phylogenetically diverse RT by NRTI and NNRTI drugs and by RNA pseudoknot aptamers.” J. Virol.81:5375-5384 (featured in JVI Spotlight).
- Jay D. Kissel, Daniel M. Held, Richard W. Hardy & Donald H. Burke (2007) “Active site binding and sequence requirements for inhibition of HIV-1 reverse transcriptase by the RT1 Family of Single-Stranded DNA Aptamers.” Nuc Acids Res 35(15):5039-50. Epub 2007 Jul 21.
- Daniel Held, Jay Kissel, James Patterson, David G. Nickens & Donald H. Burke (2006) “Aptamer inhibitors of HIV-1 proteins.” Frontiers in Bioscience special issue on RNA Therapeutics Targeted to HIV Jan 1,11:89-112.
- Daniel M. Held, Jay D. Kissel, Dayal Saran, Daniel Michalowski, and Donald H. Burke (2006) “Differential susceptibility of HIV-1 reverse transcriptase to inhibition by RNA aptamers in enzymatic reactions monitoring specific steps during genome replication.” J. Biol. Chem. 281:25712-25722.
- James Patterson, David G. Nickens & Donald H. Burke (2006) ” HIV-1 reverse transcriptase pausing at bulky 2′ adducts is relieved by deletion of the RNase H domain.” RNA Biology 3:163-169
RNA Catalysis-related Publications, 2005-2012
- Elisa Biondi & Donald H. Burke. (2012) “Analysis of sulfur-containing RNAs using organomercurial gels.”
Methods Mol Biol. 2012;883:111-20.
- Elisa Biondi, Adam W. R. Maxwell & Donald H. Burke. (2012) “A small kinase ribozyme with dual-site activity.” Nucleic Acids Res. 2012 Aug;40(15):7528-40. doi: 10.1093/nar/gks356. Epub 2012 May 21.
- Elisa Biondi, Andrew W. Sawyer, Adam W. R. Maxwell, and Donald H. Burke. “A dual-site acting kinase ribozyme with a highly unusual dependence on both Cu2+ and pH.” (Submitted).
- Donald H. Burke, Sugata Roychowdhury & Manami Roychowdhury-Saha (2011) “Conformational heterogeneity and tertiary stabilization in the hammerhead ribozyme from the cave cricket Dolichopoda” RNA Biology Sept 1; 8(5). (Epub ahead of print).
- Elisa Biondi, David G. Nickens, Dayal Saran Samantha Warren & Donald H. Burke (2010) “Convergent donor and acceptor substrate utilization among members of a new family of kinase ribozymes ” Nucleic Acids Res. 38:6785-6795. Epub 2010 May 28.
- David G. Nickens*, Nirmala Bardiya*, James T. Patterson, Steven R. Rhee & Donald H. Burke (2010) “Template-directed ligation of tethered mononucleotides for kinase ribozyme selection” PLoS One. 2010 Aug 24;5(8). pii: e12368.
- Donald H. Burke & Steven S. Rhee “Assembly and Activation of a kinase ribozyme.” (2010) RNA 16:2349-59. Epub 2010 Oct 8.
- Dayal Saran & Donald H. Burke (2007) “Synthesis of photo-cleavable and non-cleavable substrate-DNA and substrate-RNA conjugates for the selection of nucleic acid catalysts.” Bioconj. Chem., 18:275-279.
- Manami Roychowdhury-Saha & Donald H. Burke “Monovalent cations promote a distinct reaction pathway for substrate cleavage in tertiary stabilized hammerhead ribozymes.” RNA 13:841-848.
- Dayal Saran, Daniel M. Held & Donald H. Burke (2006) ” Multiple-turnover thio-ATP hydrolase and phospho-enzyme intermediate formation activities catalyzed by an RNA enzyme.” Nuc. Acids Res. 34: 3201–3208.
- Manami Roychowdhury-Saha & Donald H. Burke (2006) “Extraordinary rates of transition metal ion-mediated catalysis by a tertiary stabilized hammerhead ribozyme.” RNA 12:1846-52.
- Bong-Rae Cho & Donald H. Burke (2006) “Structural stabilization of the Kin.46 self-kinasing ribozyme through topological rearrangement.” RNA 12:2118-25.
- Donald H. Burke & S. Travis Greathouse (2005) “Low-magnesium, trans-cleavage activity by type III, tertiary stabilized hammerhead ribozymes with stem 1 discontinuities.” BMC Biochemistry 6:14.
- Dayal Saran, David G. Nickens & Donald H. Burke (2005) “A trans acting ribozyme that phosphorylates exogenous RNA.” Biochemistry 44:15007-15016.
Other Recent Publications 2002-2004
- Steven Rhee & Donald H. Burke (2004) “Tris(2-carboxyethyl)phosphine (TCEP) stabilization of RNAs: comparison with dithiothreitol (DTT) for use with nucleotide and thiophosphoryl chemistry.” Analytical Biochemistry 325:137-143.
- Donald H. Burke (2004) “Ribozyme-catalyzed genetics” Chapter 4 of Genetic Code and the Origin of Life, ed Lluis Ribas de Pauplana.
- Vanvimon Saksmerprome, & Donald H. Burke (2004) “Deprotonation stimulates productive folding in allosteric TRAP hammerhead ribozymes.” Journal of Molecular Biology 341:685-694.
- Vanvimon Saksmerprome, Roychowdhury-Saha M., Anastasia Khvorova, Sumedha Jayasena & Donald H. Burke (2004) ” Artificial tertiary motifs stabilize trans-cleaving hammerhead ribozymes under conditions of submillimolar divalent ions and high temperatures.” RNA 10:1916-1924.
- Dayal Saran, Joseph Frank, & Donald H. Burke (2003) “The tyranny of adenosine recognition among aptamers to coenzyme A.” BMC Evol. Biol. 3:26.
- Daniel Held, S. Travis Greathouse, Amit Agrawal, & Donald H. Burke (2003) “Evolutionary landscapes for the acquisition of new ligand recognition by RNA aptamers.” Journal of Molecular Evolution 57:299-308
- David Nickens, James T. Patterson & Donald H. Burke (2003) “Inhibition of HIV-1 reverse transcriptase by RNA aptamers in Escherichia coli .” RNA 9:1029-1033.
- Vanvimon Saksmerprome & Donald H. Burke (2003) “Structural flexibility and thermodynamics of helix exchange constrain allosteric activation of hammerhead ribozyme TRAPs.” Biochemistry 42: 13879-86.
- Sanchita Hati, Amy R. Boles, Amanda Posto, John Zaborske, Brett Bergman, and Donald H. Burke (2003) “Nickel 2+-mediated assembly of RNA-amino acid complexes.” Chem. & Biol. 10: 1129-37.
- Manami Roychowdhury-Saha, Susan M. Lato, Eric D. Shank, & Donald H. Burke (2002) ” Flavin recognition by an RNA aptamer targeted toward FAD.” Biochemistry, 41:2492-2499.
- Susan M. Lato, Nicole D. S. Ozerova, Kaizhang He, Zinaida Sergueeva, Barbara Ramsey Shaw, & Donald H. Burke (2002) “Boronated RNA aptamers to ATP.” Nuc. Acids Res. 30:1401-1407.
- Donald H. Burke, Nicole D.S. Ozerova, & Marit Nilsen-Hamilton (2002) “Allosteric hammerhead ribozyme TRAPs.” Biochemistry 41:6588-6594.
- Donald H. Burke & David Nickens (2002) “Expressing RNA aptamers inside cells to reveal proteome and ribonome function.” Briefings in Functional Genomics and Proteomics 1:169-188